SNOW CHEMISTRY ACROSS ANTARCTICA - page 4
Ion Concentration versus elevation (continued)
No statistically significant correlation at the 99.9‰ significance level exists between elevation and NO3, (Fig. 2d). NO3 is predominantly a secondary aerosol, produced in the strato- and ionosphere. Processes leading to nitrate production in the higher atmosphere are thought to be stratospheric oxidation of N2O, ionospheric dissociation of N2, and polar stratospheric clouds via HNO3. Additionally, lightening in the mid-latitudes produces the primary NO3 aerosol in the troposphere (Parker and others, 1977; Parker and Zeller, 1980; Parker and others, 1981; Parker and others, 1982; McKenzie and Johnston, 1984; Evans and others, 1985; Legrand and Delmas, 1986; Toon and others, 1986; Legrand and Kirchner, 1990; Mayewski and others, 1990; Qin and others, 1992; Solomon and Keys, 1992; Clausen, 1995; Wolff, 1995; Yang and others, 1996a; Yang and others, 1996b; Legrand and Mayewski, 1997; Wagenbach and others, 1998b; Palmer and others, 2001). Higher NO3 concentrations are therefore expected within the boundary o of the polar vortex due to the influence of upper atmospheric air masses. Furthermore, some post-depositional and photochemical mechanisms lead to NO3 loss, especially at low accumulation sites (De Angelis and Legrand, 1995; Legrand and Mayewski, 1997; Mulvaney and others, 1998; Wagnon and others, 1999; Röthlisberger and others, 2000; Jones and others, 2001; Röthlisberger and others, 2002; Wolff and others, 2002; Udisti and others, in press), which partially offsets the trend towards higher NO3 in the Antarctic interior. As this effect takes place in the upper few meters of the snow pack it is particularly important not only to compare contemporary NO3 data, but also samples derived from similar depths in the snow profile.
The correlation between SO4 and elevation also shows no statistically significant trend at the 99.9‰ significance level (Table 1). While concentration data exhibit a more scattered pattern at lower elevations, total SO4 input seems largely independent from elevation. However, SO4 has many sources (Delmas and others, 1982; Wolff and Mulvaney, 1991; Mayewski and others, 1992; Mulvaney and Wolff, 1994; Legrand, 1995; Legrand and Mayewski, 1997; Minikin and others, 1998; Udisti and others, 1998; Udisti and others, 1999; Becagli and others, in press) and individual SO4 species might exhibit significant correlations with elevation. While primary aerosol SO4 species (sea spray) and secondary marine-biogenic SO4 should exhibit a rapid decrease with increasing elevation, volcanic SO4 aerosols enters through the upper atmosphere and therefore should have a stronger signal in the Antarctic interior. Furthermore, the volcanic input of SO4 often exceeds average SO4 input (Mayewski and others, 1995; Zielinski and others, 1997; Dixon and others, Annals, 41). Because data used in this comparison represent different time periods, samples containing volcanic SO4 input have the potential to obscure an existing relationship, especially for the 1992 to 1997 time period, which coincides with the Mount Pinatubo 1991 eruption.
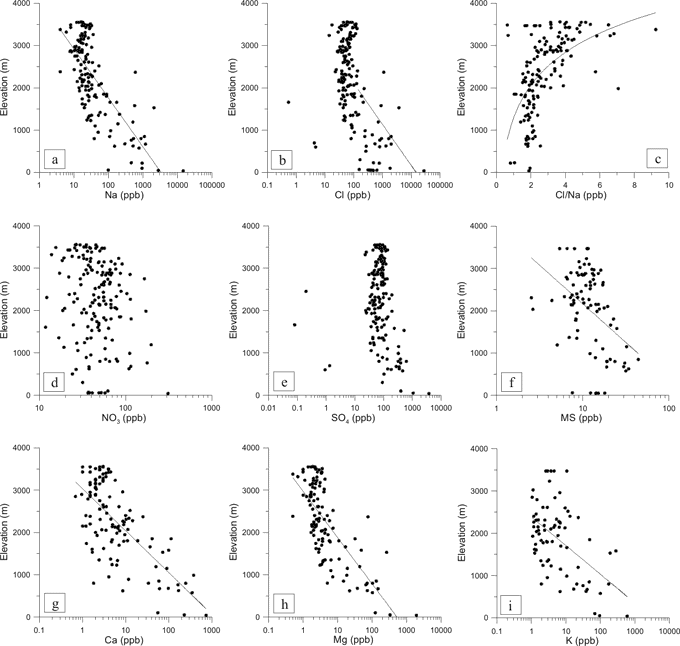
Figure 2: Relationship between multi-year ion concentration data and elevation. Please note, all species are plotted on logarithmic scale,with the exception of Cl to Na ratio, which is plotted on a linear scale. The logarithmic trends shown are significant on the 99.9% level.
MS shows a statistically significant decrease with increasing elevation (Fig. 2f) of r = -0.42 (Table 1). While most ion species have multiple sources, MS is thought to have a single marine source and is derived via oxidation from plankton-produced DMS (Dimethylsulfonate) in polar oceans (Mulvaney and others, 1992), which explains its anti-correlation with elevation. MS is observed to peak in summer, when biological activity is highest (Welch and others, 1993; Saltzman, 1995; Saltzman and others, 1997; Meyerson and others, 2002). Delmas and others (2003) and Wellers and others (2004) describe a mechanism by which MS is lost to interstitial gaseous phase in the Antarctic interior, which might partially be responsible for the observed trend in Fig. 2f. As for NO3 it is therefore important to investigate MS data in relationship to snow depth of the sample.
Ca, Mg, and K show an inverse relationship with decreasing concentration at higher elevation sites (Fig. 2g, h) with r = -0.70 and r = -0.73, r = -0.52 respectively. Furthermore, the scatter in the data sets appears higher at lower elevations sites, especially for Ca. These species have local and global terrestrial, as well as marine sources. In the vicinity of ice-free areas, such as the McMurdo Dry Valleys, ion concentration is influenced from those local sources (Gayley and Ram, 1985; Aristarain and Delmas, 2002; Bertler and others, 2004b). Elsewhere, the input is dominated by sea-salt and global dust input (Shaw, 1979), with the former producing orders of magnitude higher concentrations than the latter, thus explaining the overall inverse relationship with elevation.